Osteogenesis, or new bone formation, begins in the early stages of embryonic development and continues postnatally until skeletal maturity is reached in early adulthood. Osteogenesis reoccurs in the event of bone trauma, such as a fracture or surgically created defect. Bone mass can also increase in response to chronic weight bearing from daily use or decrease due to a lack of movement or applied force, further illustrating the dynamic nature of bone tissue. A closer look at the biological processes that drive osteogenesis, particularly in the embryonic environment, can provide insights into the mechanisms of normal bone remodeling and clinically-assisted bone regeneration.
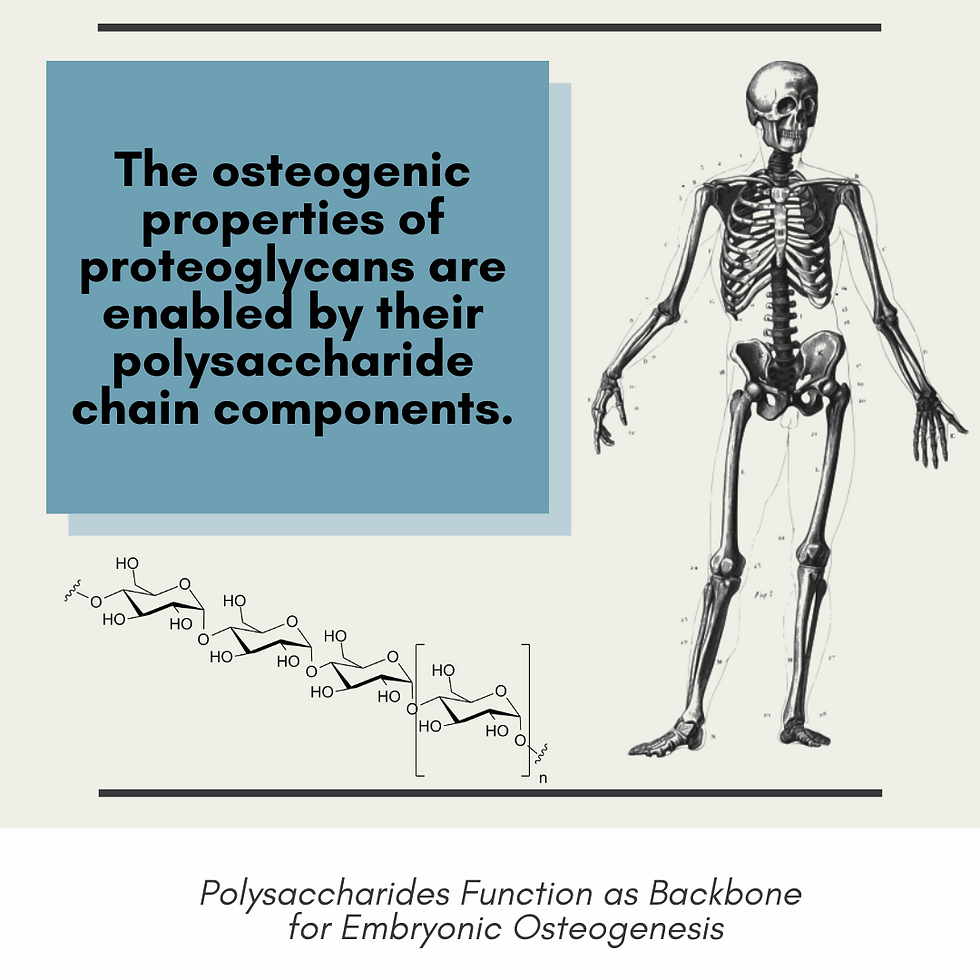
Two processes result in bone development in the embryonic environment: endochondral ossification and intramembranous ossification (Gilbert 2000). Endochondral ossification, which forms the long bones of the appendicular skeleton, occurs within a cartilaginous skeleton template that becomes bone over time. The cartilage template is composed of chondrocyte cells that produce a matrix of collagen, elastin, and polysaccharides. When chondrocytes stop producing this extracellular matrix and the template is complete, mineral deposition begins to calcify the template. Bone-forming osteoblasts infiltrate the calcified template and populate its cavities with mature bone cells, or osteocytes. Intramembranous ossification is the direct production of bone tissue without a cartilage template, and ultimately forms the skull and vertebrae of the axial skeleton (Gilbert 2000). Intramembranous ossification takes place within the mesenchyme, an early form of connective tissue resembling the collagenous extracellular matrix (ECM). Similar to the ECM, the mesenchyme houses a range of cells, proteins, and polysaccharides that are involved in bone development.
The ECM provides essential structural and biochemical support during embryonic osteogenesis. The structure of the ECM is composed of an interlocking mesh of proteins and long, unbranched polysaccharides called glycosaminoglycans (Raspanti et al. 2008). The majority of proteins are covalently attached to glycosaminoglycan chains, thereby forming proteoglycans. Proteoglycans are considered a class of proteins although they more closely resemble polysaccharides due to their 95% glycosaminoglycan composition. Types of proteoglycans are further classified on the basis of the glycosaminoglycan subtype they contain as opposed to their protein core. Proteoglycans have diverse functions in cartilage and bone, and display osteogenic properties contributing to embryonic osteogenesis (Iozzo and Murdoch 1996). Proteoglycans are expressed during both endochondral and intramembranous ossification and are concentrated in areas of active osteogenesis (Sugars et al. 2013). In cartilaginous tissues such as the early skeleton template, proteoglycans help form a stable matrix capable of withstanding compressive forces without losing structural integrity (Iozzo and Schafer 2015). Within the ECM, proteoglycans interact with other proteins, such as integrins, to facilitate cellular infiltration, cell to cell interaction, and cellular adhesion throughout the matrix. Certain proteoglycans have been found to bind and modulate the bioactivity of growth factors, including bone morphogenetic proteins, that are directly involved in the differentiation of chondrocytes and osteoblasts (Decarlo et al. 2012). Numerous studies show that proteoglycan deficiencies result in reduced osteoblast populations and activity, impaired bone formation, and failure to reach peak bone density at maturity compared to control organisms (Iozzo and Schaefer 2015).
Bone homeostasis in the mature skeleton involves a balance between the function of bone-forming osteoblasts and bone-dissolving osteoclasts. During bone remodeling in the mature skeleton as well as during osteogenesis in the embryonic environment, osteoblast activity is upregulated to produce new bone cells and osteoclast activity is downregulated to reduce the loss of existing bone. Research suggests that proteoglycans can promote osteoblast differentiation and suppress osteoclast activity, thereby enhancing the biological drivers of osteogenesis. Various organic and synthetic materials (e.g., autologous bone, allogenic bone, metals, ceramics, polymers, and growth factors) are used clinically to promote bone regeneration in patients with bone voids or defects, though proteoglycans are not (Kim et al. 2020). Proteoglycans are difficult to synthesize and isolating endogenous proteoglycans in efficacious quantities is impractical. Administering molecules in this treatment modality also poses significant issues due to their rapid degradation and potential for immunogenicity. Furthermore, biologics typically require a carrier material for surgical implantation in order to deliver the molecules to the intended treatment site, retain the molecules at the site, and prevent leaching into surrounding tissues and the bloodstream.
It is believed that the polysaccharide chain component of proteoglycans, as opposed to the protein core, is the component responsible for its osteogenic properties. For this reason, a carbohydrate-based implant that utilizes a polysaccharide backbone may be an effective alternative while also eliminating the risks associated with bioactive molecules. Carbohydrate polymer materials possess structural similarities to glycosaminoglycans and may mimic their role in bone regeneration. Preclinical studies indicate that one such implant material, a hyper-crosslinked carbohydrate polymer (HCCP) scaffold, was effective in promoting bone healing in a surgically-created defect. HCCP can be synthesized with relative ease and can provide a biocompatible osteoconductive environment for bone regeneration (Koleva et al. 2019).
References
Decarlo AA, Belousova M, Ellis AL, et al. Perlecan domain 1 recombinant proteoglycan augments BMP-2 activity and osteogenesis. BMC Biotechnol. 2012;12:60.
Gilbert SF. Developmental Biology. 6th edition. Sunderland (MA): Sinauer Associates; 2000. Osteogenesis: The Development of Bones.
Iozzo RV, Murdoch AD. Proteoglycans of the extracellular environment: clues from the gene and protein side offer novel perspectives in molecular diversity and function. FASEB J. 1996;10(5):598-614.
Iozzo RV, Schaefer L. Proteoglycan form and function: A comprehensive nomenclature of proteoglycans. Matrix Biol. 2015;42:11-55.
Kim T, See CW, Li X, Zhu D. Orthopedic implants and devices for bone fractures and defects: Past, present and perspective. Engineered Regeneration. 2020;1:6-18.
Koleva PM, Keefer JH, Ayala AM, et al. Hyper-Crosslinked Carbohydrate Polymer for Repair of Critical-Sized Bone Defects. Biores Open Access. 2019;8(1):111-120.
Raspanti M, Viola M, Forlino A, Tenni R, Gruppi C, Tira ME. Glycosaminoglycans show a specific periodic interaction with type I collagen fibrils. J Struct Biol. 2008;164(1):134-139.
Sugars RV, Olsson ML, Marchner S, Hultenby K, Wendel M. The glycosylation profile of osteoadherin alters during endochondral bone formation. Bone. 2013;53(2):459-467.
Comments